Hardwood Ecology
Introduction
An understanding of hardwood ecology is necessary for successful management. A lack of ecological understanding often results in costly delays or failures in meeting specific management objectives. Ecological understanding begins with an appreciation of the functions and values of hardwood ecosystems and proceeds to the five “S” factors of hardwood ecology: site, species, species-site relationships, succession, and stand development. Recognizing different sites and species occurring in hardwood forests is an important first step toward successful hardwood management. Integrating these factors into species-site relationships is the next step. Integration begins the complicated system of hardwood management. This step is followed by understanding how these species-site relationships change over time through successional processes of site, species, and their interactions. Finally, understanding hardwood ecology must include knowing the interaction of individual species in competition or stand development. This publication reviews each of these aspects of hardwood ecology.
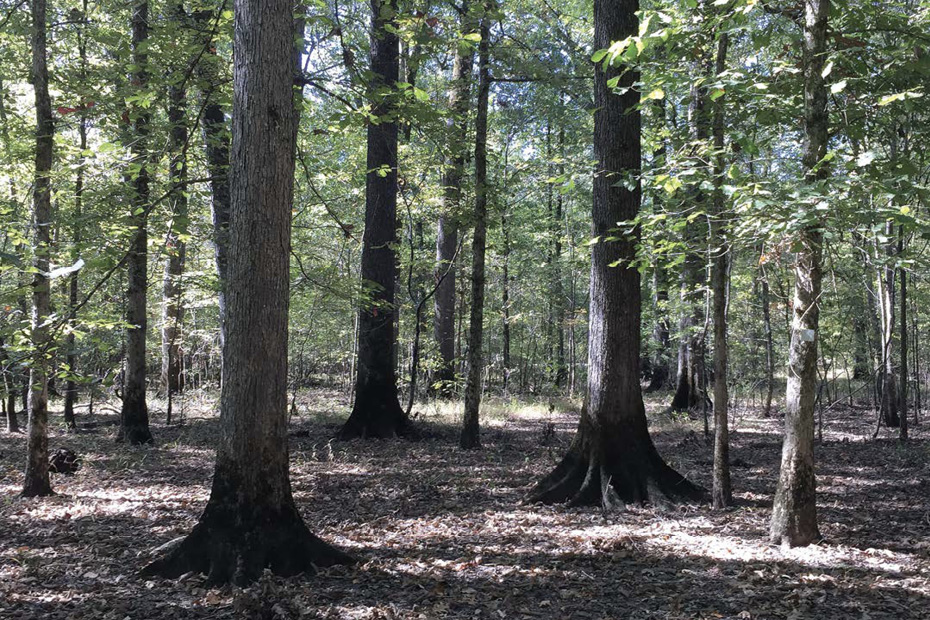
Bottomland hardwood ecosystems have long been thought of in terms of their usage. Only in the past 30 to 40 years have functions of bottomland hardwood ecosystems been given significant consideration. Early efforts to study bottomland hardwood ecosystems often interchanged function and value. Today, however, these ecosystems are viewed both as the functions they conduct and the values they serve. Bottomland hardwood functions are defined as the natural activities that take place in forested wetlands—specifically, the things that forested wetlands do. Bottomland hardwood values refer to benefits derived from goods and services resulting from functions that forested wetlands perform (Figures 2 and 3). Although not an exhaustive list, this publication provides many functions and values that forested wetlands can provide. It is important to note that, while values may be inherently desirable, without consideration of functions, management of these ecosystems may be impossible.
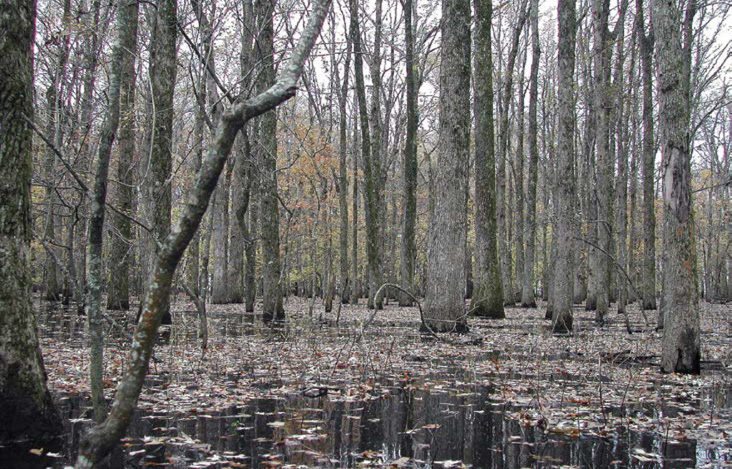
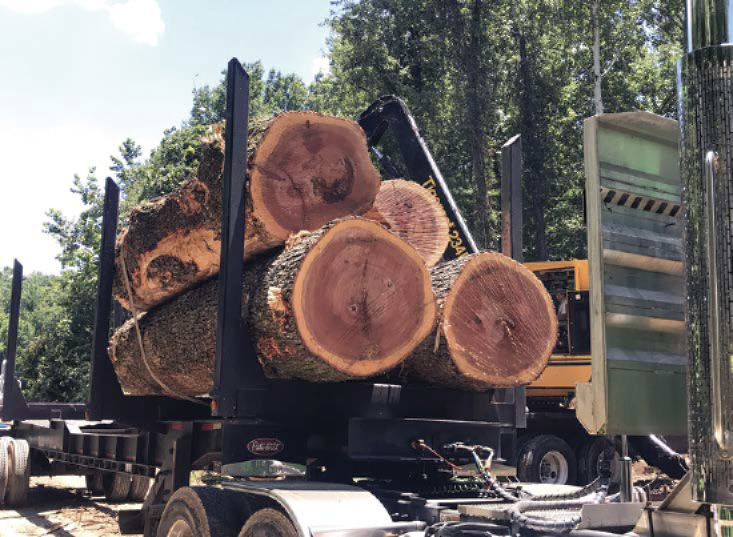
Functions and Values of Bottomland Hardwoods
Functions
- Hydrologic flux and storage
- aquifer (ground water) recharge to wetland and/or discharge from the ecosystem
- water storage reservoir and regulator
- regional stream hydrology (discharge and recharge)
- regional climate control (evapotranspiration export equals large-scale atmospheric losses of water)
- Biological productivity
- net primary productivity
- carbon storage
- carbon fixation
- secondary productivity
- Biogeochemical cycling and storage
- nutrient source or sink on the landscape
- C, N, S, P, etc., transformations (oxidation/reduction reactions)
- denitrification
- sediment and organic matter reservoir
- Decomposition
- carbon release (global climate impacts)
- detritus output for aquatic organisms (downstream energy source)
- mineralization and release of N, S, C, etc.
- Community/wildlife habitat
- habitat for species (unique and endangered)
- habitat for algae, bacteria, fungi, shellfish, wildlife, and wetland plants
- biodiversity
Values
- Flood control (conveyance), flood storage (1, 2)*
- Sediment control (filter for waste) (3, 2)
- Wastewater treatment system (3, 2)
- Nutrient removal from agricultural runoff and wastewater systems (3, 2)
- Recreation (5, 1)
- Open space (1, 2, 5)
- Visual/cultural (1, 5)
- Hunting (5, 2)
- Preservation of flora and fauna (endemic and refuge) (5)
- Timber production (2, 1)
- Shrub crops (berries) (2, 1)
- Medical (5, 4)
- Education and research (1–5)
- Erosion control (1, 2, 3)
- Food production (shrimp, fish, and ducks) (2, 5)
- Historical, cultural, and archaeological resources (2)
- Threatened, rare, and endangered species habitat (5)
- Water quality (3, 1)
- Water supply (1)
* Denotes values that are directly related to functions 1–5, or those functions that can be adversely affected by overutilization of values. The order of the numbers suggests which primary function is most directly or first affected.
Hardwood Sites
A site is simply the area in which a tree or forest stand grows. It represents the sum of environmental factors (e.g., soils, topography, and climate) that affect quality and quantity of vegetative growth. One fundamental building block for properly understanding the ecological interactions of hardwood species is knowing the various factors that influence any given site. Site evaluation for forest management is important for several reasons:
- Setting objectives based on site suitability for specific plant and animal species;
- Determining a site’s suitability for recommending specific tree species for planting efforts;
- Predicting quality and quantity of timber production;
- Predicting yield of wildlife habitat quality and quantity, including browse, hard mast, and soft mast production; and
- Assessing impacts of land use activities, such as timber harvesting, on future production of desired products.
Site Quality
Site quality is perhaps the most important characteristic in hardwood management. Characteristics representative of “good” hardwood sites are more specific than for pine sites due to greater nutrient demand of hardwoods. Furthermore, hardwood species tend to be more susceptible to the effects of stress (reduced diameter growth, epicormic branch production, crown dieback, and mortality), especially for water and nutrients, compared to conifer species. Tables 1 and 2 provide basic delineation of sites by their type and characteristics using site quality.
Site quality |
Type of site |
---|---|
High |
|
Low |
|
Site quality |
Type of site |
---|---|
High |
|
Low |
|
Site Classification
All topographic positions in Mississippi can be classified as either upland, floodplain, or terrace. Other than relief, the major differences between these sites is origin of the soils. Upland sites are characterized by soils formed in place by weathering of geologic materials. Soils formed in place and by downslope movement from upslope positions (colluvium soils) are also characteristic of specific upland site types. The Brown Loam Bluffs (also called loessal hills), developed from wind-deposited silts, are also an important upland hardwood site in Mississippi. Floodplain and terrace sites usually contain younger soils than upland sites. Soils in floodplains were formed after stream deposition of sediments (alluvium). Floodplains are made up of relatively young soils. Terrace sites are older, higher floodplains that do not flood any longer. Upland hardwoods typically occur on upland sites, while bottomland hardwood species occur primarily on alluvial floodplain sites. A mixture of these species can be found in the Brown Loam Bluffs and on terrace sites—reflecting the transitional nature of terraces between uplands and floodplains.
Floodplain Location, Origin, and Formation
Hardwood sites are found throughout Mississippi from the upland Appalachian foothills in northeast Mississippi to alluvial floodplains along most streams and rivers. They are most common in regions where soils are derived from poorly consolidated, easy erodible sedimentary material and where streams have lower gradients with low water velocity during floods.
Geographically, stream valleys of the coastal plain may be quite old. However, most floodplain sediment, and sometimes younger terrace deposits, were formed in recent geologic times. The origin of the Mississippi Alluvial Plain (MAP), also known as the Mississippi Delta, is different from that of other Coastal Plain floodplains. This is primarily due to effects of glaciation on the landscape, as well as the large volume of water and sediment that has historically moved through the area. During glacial periods, the MAP received thick deposits of sediment that were eroded by water from glacial melt and/or very heavy rainfall over time. In recent times, flow has been much lower, and the region is still gaining elevation through sediment deposition. However, the system has been altered through construction of the present levee system. Meandering of the Mississippi River across the broad floodplain resulted in the formation of a diverse topography composed of natural levees, ridges, backwater swamps (flats), and sloughs.
River valleys have an active floodplain and terrace system, often with three or more terraces. Terraces (older floodplains) are not typically as productive for bottomland hardwood growth as current floodplains, but they are important for hydrologic control over the modern floodplain. Except for very young terraces, they rarely flood.
Two theories have been noted regarding the origins of stream terraces: 1) downcutting and lateral movement of the present stream, and 2) changes in external forces, such as earth movement, which influence deposition and erosion. During downcutting, the river cuts vertically and laterally as it moves across the floodplain. This process assumes sediment removal on the floodplain exceeds deposition. The more likely theory is that typical terrace formation is related to changes in external forces. Marked increases in rainfall could result in intensified erosion, thus lowering the floodplain. At this point, portions of the floodplain remaining at the original elevation become terraces. Another process for terrace formation would be a series of valley fillings by oceanic or river deposition with subsequent erosion of the deposition.
Strong evidence supporting the influence of climatic change on stream valleys and floodplains is shown in the occurrence of “underfitted” streams in the Coastal Plain. In such valleys, current streams are far too small in terms of flow and dimension to account for floodplain width. The most logical explanation for formation of these floodplains is that water discharge into the valley was much higher in prehistoric times. Research shows that current floodplains are young surfaces where erosion and deposition are taking place with deposition being the primary process. Most floodplains have variations in topography that results from stream movement and sediment deposition (Figure 4). When streams overflow their banks, water velocity immediately starts to slow and larger soil particles start to settle. This results in rapid deposition forming natural levees or “fronts” near the stream edge. Fronts are often the highest elevation landform in the floodplain. Slower rates of deposition occur on flats due to very slow water movement. As a stream meanders, new fronts are formed, and old fronts become ridges within the floodplain. The old stream channel typically becomes a lake, eventually filling with sediment and turning into swamps and/or sloughs. Stream movement can also result in the formation of “ridge and swale” topography. These ridges are formed as point bars, usually have sandy soils, and are usually narrower than ridges. Features described previously may not occur in younger and smaller stream bottoms where stream meandering has not occurred.
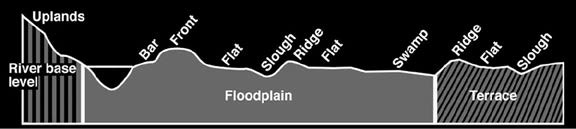
Types of Floodplains
Bottomlands are often classified as either “major” or “minor” floodplains, or as “bottoms.” The obvious difference between the two is stream size; major floodplains have larger streams (and a larger drainage basin) than minor floodplains. However, another, more crucial difference has to do with the source and nature of the sediment deposited. In major floodplains, sediment may be transported for hundreds of miles, vary greatly in mineralogy, and be a mixture of all textural classes. In minor floodplains, sediment is of local origin and is usually not as deep as in major floodplains. For these reasons, major floodplains are generally more productive than minor floodplains. In Mississippi, major floodplains are associated with the Mississippi River and its associated tributaries in the MAP. These include the Yazoo, Sunflower, Little Sunflower, and Tallahatchie Rivers. Other major floodplains are associated with the Pearl, Pascagoula, and Tombigbee (Tennessee-Tombigbee Waterway) Rivers. Remaining rivers and streams are classified as minor floodplains.
Floodplain Topography
Site variation within floodplains is primarily a function of elevational differences. Differences of only 1 to 3 feet often have drastic effects on site characteristics and species occurrence. Elevational changes primarily reflect differences in drainage and soil moisture, but minor elevational variance also results in differences in soil type, texture, structure, and pH. These can all affect species occurrence. Topographic/site variation within a floodplain is the result of stream movement and subsequent sediment deposition patterns.
Figure 4 represents a hypothetical stream valley cross section. Each topographic feature may occur several times and not necessarily in the order shown. Bars are formed when the concave section of streambanks erode, and sediment is deposited downstream on an opposite convex area of the stream channel. With time and increased deposition, the bar may become a flat and eventually rise to the level of the current front. As previously discussed, fronts are formed when streams overflow their banks and rapid deposition occurs. These sites are usually the highest, best drained, and most productive areas in the floodplain. Flats are broad, usually level areas between ridges or between ridges and fronts. Soils are predominantly clays, and the drainage class usually varies from poorly drained to somewhat poorly drained, but water does not typically stand during most of the growing season. There may be slight elevational and drainage differences on these flats even though the soils are classified within a series, accounting for terms like “high flats” and “low flats.” Flats account for the largest area within the floodplain. Ridges can vary in elevation from 2 to 15 feet above flats, but 2 to 5 feet is more common. Ridges were formed as fronts of older streams. Soils are of a coarser texture than those of flats, and drainage is better. Sloughs are shallow depressions arising from old streambeds almost filled with sediment. The soils are usually fine textured, drainage is poor, and typically water stands well into the growing season. Swamps are larger depressions formed when streams change and form lakes that continue to fill with fine-textured sediment. Drainage is very poor, and standing water is always present except in extremely dry years.
Soils
Soils and Soil Formation
Floodplains — Soils of current floodplains are young and typically exhibit little profile development. Most have been formed (deposited) in recent geologic times (last 12,000 years). An exception may occur where a current floodplain overlies older terrace materials. Soil variation may be more pronounced on floodplains with highly variable topography. Soils on fronts tend to be coarser and vary from well-drained to somewhat poorly drained. Ridge soils are often of similar texture, but they are wetter with possibility of some nutrient leaching. Soils are generally clay loams or clays on flats and vary from somewhat poorly drained to poorly drained. Soils in sloughs are typically very clayey and very poorly drained. Expansive clays (smectites) are common on floodplains, especially MAP and Gulf Coastal Plain sites. Within the floodplain, site productivity and plant biodiversity tend to decrease in this order: front > ridge > flat > slough.
Terraces — Terrace soils are older than floodplain soils, and horizons tend to be well developed. Terrace sites often support a variety of hardwood species, but growth and quality are generally not as good as that of floodplain sites. This difference is attributable to leaching of nutrients, development of fragipans (restrictive layer that can inhibit root penetration), and less favorable soil moisture relationships.
Loessal Uplands — The Brown Loam Bluffs are potentially the most productive hardwood sites in the world (Figure 5). Researchers noted that these soils were developed from wind-deposited silts between 12,500 and 40,000 years ago. The loess forms a thick mantle ranging in thickness from 2 feet to more than 100 feet over unconsolidated sands, sandy clays, and gravels. These soils are brown silt loams, well drained, and moderately permeable, but highly erosive. Soil pH varies from near neutral on lower slopes and bottoms to strongly acidic on ridgetops, especially those with a history of agricultural use.
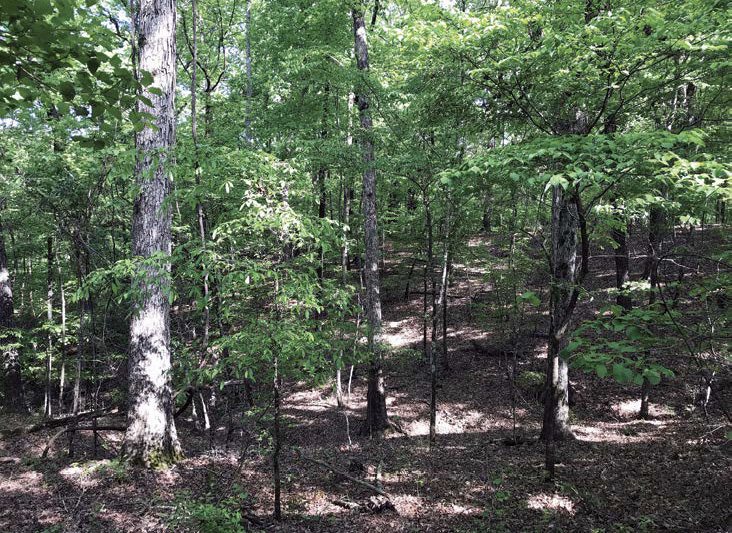
Species
More than 70 tree and woody shrub species are found in bottomland and upland hardwood sites in Mississippi. Many of these species have commercial and/or wildlife habitat value. Identifying tree and shrub species along with a thorough understanding of their silvical characteristics is the second step in managing hardwoods. While an in-depth understanding of many of these characteristics may require an experienced professional forester, understanding the general concept is not too difficult.
Shade Tolerance
Shade tolerance refers to the ability of a species to tolerate shade from competing vegetation. Categories range from very intolerant, meaning the species cannot tolerate any shade, to very tolerant, meaning the species can thrive in the understory of other trees. It should be noted that most desirable species managed in hardwood forests, especially for timber production, tend to be shade intolerant or moderately shade intolerant.
Flood Tolerance
By the nature of their topographic position, bottomland hardwood forests are subjected to high water table levels, soil saturation, and periodic and/or continuous flooding at various times of the year. On these sites, relative flood duration and depth play key roles in occurrence and growth rate of tree species from seed germination through early survival and growth during establishment, as well as later tree growth. Survivability of a tree on these wet sites depends on a combination of inherent biological traits and adaptive characteristics related to flood tolerance. Bottomland species differ in flood tolerance and tree characteristics, each having influence on their occurrence across the forest. Several flood tolerance categories exist, ranging from tolerant to intolerant. Tolerant species can survive and grow on sites where soils are saturated or flooded for long, indefinite periods during the growing season. These species have anatomical and physiological flood tolerance adaptations, allowing oxidation of their rhizosphere (soil region immediately surrounding plant roots) and control of anaerobic respiration in roots during periods of low oxygen levels and the resulting toxic compounds.
Moderately tolerant species can survive saturated or flooded soils for several months during the growing season, but mortality is high if flooding persists or reoccurs consecutively for several years. These species may develop adventitious roots (forming from any nonroot tissue) or other adaptations, but these adaptations are not sufficient for life in waterlogged soils. Weakly tolerant species can survive saturated or flooded soils for a few days to a few weeks during the growing season, but they suffer high mortality if flooding persists for longer periods. Intolerant species are not able to survive even short periods of soil saturation or flooding during the growing season.
Species/Site Relationships
Major Floodplains
Figure 6 shows a representation of species typically found on various topographic positions across the floodplain of major streams. While these species/site relationships are considered typical, variations occur depending on the successional stage of that particular system. On the newest land near the river (bar position), black willow is the major pioneer species; but if the site is high enough, or if adequate time is available between high water events, eastern cottonwood may establish. Black willow and eastern cottonwood need mineral soil for establishment, but black willow is more water tolerant. Both species can withstand sediment deposition; if deposition continues, a front site will form. In the event of front formation, both species will eventually be replaced by riverfront species.
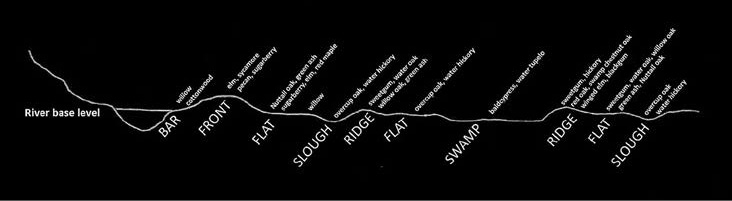
On frontal sites, a typical species association includes American elm, American sycamore, sweet pecan, sugarberry, boxelder, and sweetgum. This riverfront mixture is not the highest point, and the future species mix depends on a variety of factors. If stand breakup is rapid (human-induced or natural disaster), a red oak-sweetgum type may develop if advance oak regeneration is present. If stand breakup is slow, the stand will degenerate to a boxelder-sugarberry or boxelder-hackberry-silver maple mixture. As that stand matures and goes through its own breakup, it may eventually become one composed of sweetgum and red oaks.
Species composition on the flats is extremely variable. On lower, wetter flats, overcup oak, water hickory, and baldcypress often dominate with almost pure stands of overcup oak or baldcypress being common. On higher and better drained flats, the most common species mixture is elm-green ash-sugarberry with other intermixed species such as Nuttall oak, willow oak, and red maple present. Nuttall oak and willow oak will sometimes be major components of these stands.
On ridge sites, species composition depends on past events and treatments. The most common association is elm-green ash-sugarberry, but if advance regeneration exists and the site is opened rapidly, it can be replaced by a sweetgum-red oak mixture with water oak, willow oak, cherrybark oak, Shumard oak, and other minor species along with sweetgum. Without management, this stand type typically reverts back to elm-green ash-sugarberry through natural succession.
If sloughs are near the stream and/or sedimentation occurs rapidly, stands of quality black willow often develop. If sedimentation continues, black willow will be replaced by elm-green ash-sugarberry over time. On sites where sedimentation occurs slowly (distant from the river), overcup oak, water hickory, green ash, and persimmon most often follow black willow. The baldcypress-water tupelo type is the most common swamp/slough association of major stream bottoms. Depending on flood depth and duration, other tree species may include swamp tupelo, water-elm, water hickory, and overcup oak.
Minor Floodplains
Minor stream floodplains (Figure 7) are in many ways just a smaller version of major stream floodplains. They exhibit the same topographic features, and similar species occur there, although not necessarily on the same topographic position. Species occurring only on ridge positions in major floodplains often occur on flats in minor floodplains. River birch is the most common pioneer species on new bar sites, and diverse species mixes occur on fronts, including yellow poplar, American beech, American sycamore, sweetgum, cherrybark oak, Shumard oak, water oak, swamp chestnut oak, and several hickory species. Species mixtures on better drained flats and ridges typically include sweetgum, cherrybark oak, water oak, swamp chestnut oak, American elm, and hickories. On less wet flats, major species include overcup oak, willow oak, Nuttall oak, persimmon, green ash, sugarberry, and red maple. Species composition in minor floodplain sloughs varies depending on flood duration. Baldcypress and water elm are common where flood duration is longest, and overcup oak, water hickory, and persimmon will also occur where flooding is not severe. Swamps containing baldcypress and water tupelo do occur on floodplains of minor stream bottoms, but baldcypress is more common.
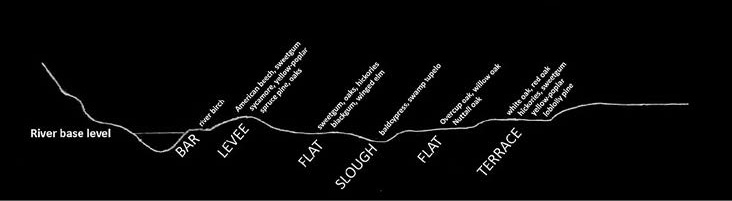
Terraces
Due to an older, well-developed soil profile, nutrient leaching, and lack of flooding deposition, terraces are less productive than active floodplains. Similar, though less distinct, topographic features occur on terraces, but species composition reflects transition from the active floodplain to uplands. While many bottomland hardwood species can be found on terraces, other species include white oak, southern red oak, black oak, and loblolly pine. Very old terraces may develop soil pans and even include post oak.
Brown Loam Bluffs
Tree species composition on the Brown Loam Bluffs is very diverse. Ridgetops, especially if previously farmed and eroded, contain typical upland species such as white oak, southern red oak, post oak, and loblolly pine. Side slopes contain a bottomland and upland species mixture including cherrybark oak, Shumard oak, white oak, sweetgum, black walnut, and yellow poplar, among others. Drainages between steep ridges often contain mixtures of sweetgum, sugarberry, American sycamore, and occasionally eastern cottonwood. Upper portions of drains will also contain various oak species. Of note, a pH gradient can occur in the Brown Loam Bluffs with highly acidic ridgetops, somewhat acidic to neutral slopes, and drainages being neutral to slightly basic. Species composition often reflects this gradient.
Succession
Succession is defined as the gradual replacement of one plant community by another. In general, two types of succession occur. Primary succession occurs on sites that lack organic matter and have not been altered by living organisms. An example is black willow or eastern cottonwood growing on recently formed sandbars. Secondary succession occurs on sites that have already been altered by plant communities. An example would be development of hardwood species underneath the initial black willow or eastern cottonwood stand. Two subtypes are recognized. Autogenic succession (caused by the plants themselves) occurs when one plant community creates an environment more suitable for establishment of other species than for itself. An example of autogenic succession involves development of eastern cottonwood stands. Shade and leaf litter produced by eastern cottonwood limits regeneration of itself; however, enough light penetrates the canopy to initiate development of advance regeneration of other species (e.g., sugarberry, green ash, and American elm). Allogenic succession occurs when changes in a plant community are caused by external forces, or disturbances, independent of the plants themselves. A common example is windthrow of trees from major wind events. In bottomland hardwood ecosystems, allogenic succession also involves flooding and deposition from the streams themselves. These external site changes, combined with changes caused by vegetation, lead to “site succession” as the site’s topography, soil texture, soil pH, and deposition patterns change. Therefore, bottomland hardwood sites are changing, typically at a very slow rate. These successional forces are related with species-site relationships. The individual species-site relationships in Figures 6 and 7 can be thought of as a moment in time, while a combination of all species-site relationships represent a snapshot of successional trends (i.e., as sites change, so will species associated with that site). Therefore, hardwood management involves an understanding of both species-site relationships and how these change over time.
Successional Pathways
Understanding successional changes in species composition is more important in bottomland hardwoods than upland hardwood systems for reasons discussed previously. Outside the loessal hills, fewer species occur in upland hardwoods compared with bottomland hardwoods, and “site succession” is often not a factor in upland hardwood management due to their slow change. Consequently, the following discussion focuses on bottomland hardwood ecosystems.
Three pathways of succession are recognized in major floodplains. One pathway occurs on permanently flooded sites such as baldcypress-water tupelo swamps. On these sites, succession is “arrested” (species composition may not change for hundreds of years). The baldcypress-water tupelo association represents the oldest tree type found in floodplain ecosystems. Stands are often 200 to 300 years old before breakup occurs.
A second pattern of succession occurs on poorly drained, low-elevation sites. Deposition on these sites is generally fine-textured and occurs at a slower rate and over a much longer period compared with better drained, higher elevation sites. Black willow is the typical pioneer species on low sites with heavy soils. On low sites, succession depends on sediment deposition rate and sometimes texture. Where little or no deposition occurs, black willow may be followed by a mix of swamp-privet, water-elm, and buttonbush. This association may be replaced by baldcypress, which may last for hundreds of years, or be replaced by overcup oak-water hickory stands if deposition is sufficient.
If deposition in willow stands occurs faster, but still relatively slowly, the site may become conducive to growing overcup oak and water hickory. If deposition continues, these sites will eventually transition to elm-green ash-sugarberry. If sedimentation is pronounced and the site gains elevation rapidly, black willow will be replaced by elm-green ash-sugarberry. An exception can occur when deposits are lighter textured (sandy or loamy) than the normal clays. Under this scenario, a temporary association composed of boxelder-sugarberry or boxelder, silver maple, and hackberry is formed. The elm-green ash-sugarberry association may also contain stems of other species such as Nuttall oak or pin oak in the northern MAP.
After site elevation increases and deposition slows, disturbances, either human-induced or natural, can result in elm-green ash-sugarberry stands being replaced by associations dominated by sweetgum and red oaks (e.g., water oak, willow oak, and Nuttall oak). This association is temporary and will revert to elm-green ash-sugarberry stands without management. Once deposition stops and soil ages, climax stands may start to appear. The first oaks appearing may be cherrybark, Shumard, and swamp chestnut, but the final climax association will also contain other white oaks and hickories. On these low-elevation sites, time required for progression from pioneer species to climax forest can be highly variable, depending on sedimentation rate. Breakup of most black willow stands begins as early as age 30, and few remnants survive beyond age 60. With slow deposition, buttonbush and cypress stands can persist for centuries. The overcup oak-water hickory type may occupy sites for 200 years or more. Where sites build rapidly, the amount of time required from pioneer to climax species will be about 600 years, mainly because boxelder-sugarberry and elm-green ash-sugarberry types are capable of self-replacement. However, major disturbance, such as windstorms, can revert these successional pathways to an earlier stage.
A third pattern of succession occurs on higher elevation, better drained ridge and front sites. Eastern cottonwood is the principal pioneer species, although black willow may occur early on some sites. Breakup of eastern cottonwood stands may begin as early as age 45, and few stems survive longer than 80 to 100 years.
Species composition following breakup of eastern cottonwood stands can be highly variable and depends on how breakup occurs and depositional patterns. Shade-tolerant species are typically well-established beneath eastern cottonwood and will capture the site if stand breakup occurs gradually. The next stand will usually be composed of boxelder and sugarberry and minor quantities of other species such as American elm and green ash. Stands in the northern portion of the state may contain hackberry as well as silver maple. These stands, especially those composed of boxelder and/or silver maple, will occupy the site for only about 60 years. They typically are replaced by elm-green ash-sugarberry stands.
If an eastern cottonwood stand breaks up rapidly (e.g., during a wind event), it may be replaced by several species, some of which are shade intolerant. Composition is often American sycamore, sweet pecan, and American elm, but species such as green ash, sweetgum, willow oak, and water oak are typically present. This association may endure for 75 to 125 years and is usually replaced by the elm-green ash-sugarberry type. However, if the stand is opened rapidly by natural catastrophe and a sufficient quantity of advance regeneration is present, it may progress to a water oak-sweetgum type. In turn, these stands would normally then be replaced by the elm-green ash-sugarberry type. The elm-green ash-sugarberry association is long lived, may replace itself, and will occupy the site for 200 to 300 years. Natural disasters may force replacement by red oak and sweetgum that may persist for 200 years or longer. Without management, these stands will revert to the elm-green ash-sugarberry type.
When flooding and sedimentation cease and soils start to mature, the climax association will begin to appear. This association may begin with species such as cherrybark, pin, and swamp chestnut oaks and take 200 years or more to progress to an oak-hickory climax. However, various disturbances, including changes of the stream channel and deposition patterns, prevent many sites from reaching the oak/hickory climax. In addition, human influences on floodplain function and topography (e.g., the Mississippi River levee system) also affect successional patterns.
In minor bottoms, river birch is often the pioneer species on new or severely disturbed lands. Soils are generally too acidic for eastern cottonwood, and if black willow occurs, it is in combination with other species. As in major floodplains, ridges, fronts, and better drained flats will support the oak-hickory climax even though flooding continues to occur.
Stand Development
Stand development involves changes in stand structure over time. An understanding of how bottomland hardwood stands develop is essential for long-term management of these forests. There are four stages of forest stand development: 1) stand initiation, 2) stem exclusion, 3) understory reinitiation, and 4) old growth. These stages assume that a major disturbance initiates development and no further major disturbances occur. The following sections are descriptions of these stages.
Stand Initiation Stage
After a major disturbance removes the existing stand, tree species begin to reoccupy the area with stems originating from one of three sources: seeds, advance regeneration, or existing stumps and roots. These new stems grow and develop, utilizing more and more available growing space until one or more necessary environmental factors become limiting (i.e., available growing space is completely utilized). In most situations, light is the first environmental factor to limit growth (occurs when crown closure occurs). After a major disturbance, species that exhibit rapid early height growth gain a competitive advantage over species exhibiting slow early height growth. This period from initial invasion by new seedlings until full occupancy occurs is referred to as the stand initiation stage. Duration of this stage varies widely and depends on multiple factors, but in the South, new stands generally fully occupy a site within 10 to 15 years after major disturbance.
Stem Exclusion Stage
Once new stems fully use available growing space and environmental factors start to limit growth, the next stage of stand development begins. This is referred to as “stem exclusion,” where new stems cannot establish in the developing stand. Due to crown closure, light conditions on the forest floor limit new stem establishment, indicating growing space is now fully used. At this point, intense competition among stems begins to occur.
In mixed-species stands, competitive ability of various species determines how they respond to competition. Early growth rate and shade tolerance are primary determinants of the competitive ability of any species during this stage of stand development. Due to variable growth and shade tolerance, species in developing stands may exhibit differences in ability to respond to this competition. Vertical stratification (separation) of individual trees and species results, and one or more species becomes dominant, suppressing growth of other species in the stand. This process is illustrated in the following scenario.
Species with rapid early height growth (pioneer species) typically dominate a stand initially. Other, more persistent species grow slowly in the understory and midstory during early stages. As this period of intense competition and stand development continues, growth rates of early dominant stems slow down, mortality increases, and these species begin to lose their dominance gradually. Consequently, more persistent species overtake pioneer species, become dominant, and suppress growth of other species in the stand. At this point in stand development, pioneer species, initially dominant, are now overtopped by the eventual dominant species. If pioneer species are shade intolerant, they gradually cease to be a component of the stand and die. Conversely, if initially dominant species are shade tolerant, they may remain growing slowly in the stand for many years. Under these conditions, these species will remain, but they constitute only a minor component of the mature stand.The overriding point of this scenario is that the eventual dominant species of the mature stand is generally not the largest or most numerous species during early stand development. Other species typically dominate at first but are overtaken as the stand develops. In many situations, the dominant species may occur infrequently during early stages of stand development and may even be overlooked as a stand component until it begins to overtop its competitors.
Understory Reinitiation Stage
As the stratified stand develops toward maturity, the overstory gradually begins to break apart as scattered trees die. Openings are created in the overstory, allowing sunlight to reach the forest floor and creating favorable conditions for development of new stems in the understory. This is the beginning of the understory reinitiation stage of stand development. Understory reinitiation may begin relatively early or late in the life of a stand. Timing is driven primarily by shade tolerance of overstory species and frequency of minor disturbances within the stand. Advance regeneration may remain in the understory for very long periods of time (sometimes 30 to 35 years for certain bottomland oak species). However, in most circumstances, advance regeneration of oaks and other moderately intolerant species live only for 5 to 10 years under a shaded understory.
Old-Growth Stage
Understory reinitiation may occur continuously within a stand over a long period of time, providing a gradual transition to the old-growth stage. This transition occurs as the mature overstory breaks up slowly through individual tree mortality. Adjacent advance regeneration in the understory is then released, and these younger stems gradually grow into the canopy. If the process continues throughout a stand over a long period, an uneven-aged, multi-strata, old-growth stand composed of trees and species of widely differing ages and sizes is formed. However, very few stands reach this last developmental stage because another major disturbance generally occurs first, sending the stand back to the stand initiation stage. Consequently, definable old-growth forest status is rarely attained, especially in areas subject to frequent major disturbances.
References
Autin, W. J., Gurns, S. F., Miller, B. J. Saucier, R. T. and Snead, J. I. (1991). Quaternary geology of the Lower Mississippi Valley. In R. B. Morrison (Ed.), Quaternary Nonglacial Geology. Conterminous U.S. The Geology of North America. v. K-2. Geological Society of America.
Challinor, J. (1932). River-terraces as normal features of valley development. Geography 17, 141-147.
Dury, G. H. (1977). Underfit streams: Retrospect, perspect, and prospect. In K. J. Gregory (Ed.), River channel changes (pp. 281-293). John Wiley and Sons.
Helms, J. A. (Ed.). (1998). The dictionary of forestry. Society of American Foresters, 210.
Hodges, J. D. (1995). The southern bottomland hardwood region and brown loam bluffs subregion. In J. W. Barrett (Ed.), Regional Silviculture of the United States (pp. 227-269). John Wiley and Sons.
Hodges, J. D. (1997). Development and ecology of bottomland hardwood sites. Forest Ecology and Management 90, 117-125.
Hodges, J. D., and Switzer, G. L. (1979). Some aspects on the ecology of Southern bottomland hardwoods. In Society of American Foresters Proceedings, North America’s forests: Gateway to opportunity, 360-365 .
Lockaby, B. G., Stanturf, J. A., and Messina, M. G. (1997). Effects of silvicultural activity on ecological processes in floodplain forests of the southern United States: A review of existing reports. Forest Ecology and Management 90, 93-100.
Meadows, J. S. (1994). Stand development and silviculture in bottomland hardwoods. In W. P. Smith and D. N. Pashley (Eds.), A workshop to resolve conflicts in the conservation of migratory landbirds in bottomland hardwood forests (pp. 12-16). USDA Forest Service General Technical Report, SO-114, 37p.
McKnight, J. S., Hook, D. D., Langdon, O. G., and Johnson, R. L. (1981). Flood tolerance and related characteristics of trees of bottomland forests of the southern United States. In J. R. Clark and J. Benforado (Eds.), Wetlands of bottomland hardwood forests. Elsevier.
Oliver, C. D., and Larson, B. C. (1996). Forest Stand Dynamics, update edition. John Wiley and Sons.
Putnam, J. A., Furnival, G. M., and McKnight, J. S. (1960). Management and inventory of Southern hardwoods. USDA Forest Service Agricultural Handbook No. 181, 102p.
Richardson, C. J. (1994). Ecological functions and human values in wetlands: A framework for assessing forestry impacts. Wetlands 14, 1-9.
Shelford, V. E. (1954). Some lower Mississippi Valley floodplain biotic communities: Their age and elevation. Ecology 35: 126-42.
Wharton, C. H., Kitchens, W. M. Pendleton, E. C., and Sipe, T. W. (1982). The ecology of bottomland hardwood swamps of the southeast: A community profile. Biological Service Program FWS/OBS-81/37, U.S. Fish and Wildlife Service, 133p.
Publication 3617 (POD-05-24)
By A. Brady Self, PhD, Associate Extension Professor, Forestry; Brian R. Lockhart, Research Forester (retired), U.S. Forest Service; and Andrew W. Ezell, PhD, Professor Emeritus, Forestry.
The Mississippi State University Extension Service is working to ensure all web content is accessible to all users. If you need assistance accessing any of our content, please email the webteam or call 662-325-2262.